Earthquakes are powerful natural events that can cause significant destruction. They occur when tectonic plates, which are massive slabs that make up the Earth’s surface, suddenly slip along faults.
The science behind earthquakes reveals that they generate seismic waves, which are vibrations that travel through the Earth and can be felt on the surface. Understanding these processes is crucial for predicting earthquakes and minimizing their impact on communities.
The magnitude of an earthquake is a measure of the energy released during the event. It is determined by the size of the fault that slips and how far the plates move.
Scientists study these factors to provide insights into the behavior of earthquakes. By examining seismic waves, researchers can learn more about what happens underground and help people prepare for the next major event.
Exploring the complex interactions between tectonic plates and the resulting seismic activity sheds light on the risks associated with earthquakes. This knowledge not only informs response strategies but also enhances public awareness about these natural phenomena.
By gaining a deeper understanding of the science behind earthquakes, individuals can better appreciate the forces that shape our planet.
Understanding Earthquake Mechanisms and Causes
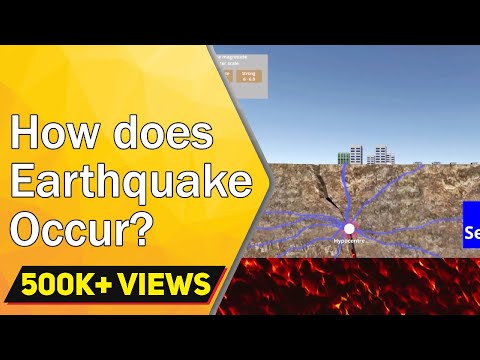
Earthquakes arise from complex physical processes involving tectonic movements, the release of seismic energy, and the structure of the Earth’s layers. This section will discuss how these aspects interconnect to explain the causes of earthquakes.
Tectonic Movements and Fault Dynamics
The Earth’s crust is divided into large sections called tectonic plates. These plates constantly move, and their interactions can create stress at fault lines. When the stress exceeds the friction holding the rocks in place, a sudden slip occurs, resulting in an earthquake.
The point where this slip happens is known as the hypocenter, while the point directly above it on the surface is the epicenter. Plate boundaries, such as those along the Ring of Fire or the mid-Atlantic ridge, are key areas where earthquakes frequently occur. Understanding these movements is essential for predicting potential seismic activity.
Seismic Waves and Energy Release
During an earthquake, energy is released in the form of seismic waves. These waves travel through the Earth’s crust, causing the ground to shake.
There are several types of seismic waves, including P-waves (primary waves) and S-waves (secondary waves). P-waves compress and expand the ground, moving the fastest. S-waves arrive later, shaking the ground up and down or side to side. The intensity and duration of shaking depend on the earthquake’s magnitude, the depth of the hypocenter, and the geological structures encountered, which can amplify or dampen the shaking.
The Earth’s Layers and Earthquake Origins
The Earth’s structure consists of several layers: the crust, mantle, outer core, and inner core. Earthquakes primarily occur in the crust and upper mantle, where tectonic plates interact.
In regions like the Alpide Belt, intense pressure builds up as plates collide, leading to explosive seismic activity. The varying composition and behavior of rocks in these layers affect how energy is stored and released during an earthquake. By studying these layers, scientists can better understand the origins of earthquakes and improve predictive models for high-risk areas.
For more on how movement affects geological structures, see this article on surface movement.
Effects and Measurements of Earthquakes
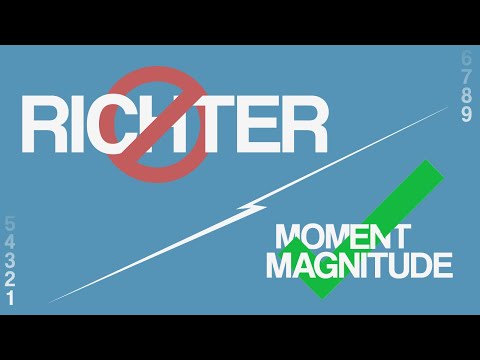
Understanding the effects and measurements of earthquakes is crucial for assessing their impact on people and the environment. This section explores how magnitude and intensity are measured, the aftermath of seismic events, and the tools used to predict and study earthquakes.
Assessing Earthquake Magnitude and Intensity
Earthquake magnitude measures the energy released during an event. The Richter scale, for example, assigns a number to this energy. Small earthquakes might register below 4.0, while major earthquakes can exceed 7.0.
Magnitude provides a uniform measurement, but intensity varies based on location and building structures. Intensity reflects the shaking experiences at different locations.
Seismic waves, which travel through the earth, include P waves (primary waves) and S waves (secondary waves). P waves travel faster and can cause initial tremors, while S waves follow and cause most of the damage. Maps showing intensity levels help identify the hardest-hit areas.
Aftermath: Aftershocks and Related Phenomena
Aftershocks occur after the main quake. They are often smaller but can still lead to damage. These secondary shocks may continue for days, weeks, or even months, gradually decreasing in frequency and size.
Additional phenomena can complicate the aftermath. For instance, landslides might happen in hilly regions, posing risks to nearby communities. Tsunamis can result from underwater earthquakes, causing severe coastal destruction. Understanding these potential aftereffects aids communities in preparing for future seismic events.
Groundbreaking Tools: Seismology and Predictive Measures
Seismology is the scientific study of earthquakes.
Seismologists use tools like seismometers to monitor and measure seismic activity. Data from these instruments is essential for determining an earthquake’s magnitude and epicenter.
Triangulation is a technique that involves using data from multiple seismometer locations to pinpoint an earthquake’s origin.
By analyzing patterns and data, scientists can improve predictive measures, allowing communities to better prepare for potential earthquakes.
Though predicting exactly when an earthquake will occur remains a challenge, advancements in seismology have made significant contributions toward understanding these natural events.