Earthquakes are mysterious yet powerful natural events that can cause significant destruction in a matter of seconds. An earthquake occurs when there is a sudden slip on a fault line, releasing energy that creates seismic waves.
This shift often results from the movement of tectonic plates, which are large pieces of the Earth’s crust that constantly shift and grind against one another.
Understanding earthquakes requires a blend of seismology and geophysics, fields that study the action of these tremors and the science behind their causes.
Scientists have been studying earthquakes for decades to better predict and understand their impact. This research helps communities prepare for potential disasters, especially in regions prone to high seismic activity.
While it is impossible to stop an earthquake, advancements in technology and knowledge of the Earth’s processes allow researchers to improve early warning systems and develop more resilient buildings.
Through careful study of the Earth’s movement, researchers can gain insights into when and where an earthquake might occur. This ongoing quest for knowledge remains crucial for reducing risks and protecting lives in earthquake-prone areas.
The Science Behind Earthquakes
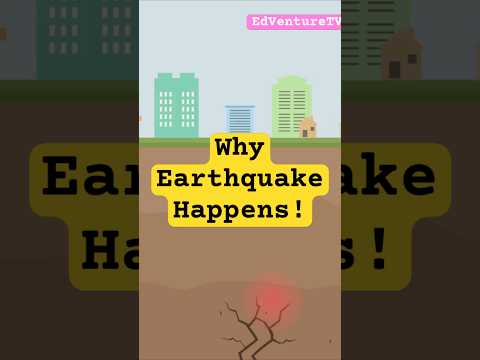
Understanding earthquakes involves examining tectonic processes, measurement, and how energy is released through seismic waves. This section explores how these factors interact and influence seismic events.
Tectonic Plate Movements
Earthquakes primarily occur due to the movement of tectonic plates. The Earth’s crust is divided into several large and small plates that float on the semi-fluid mantle beneath them.
These plates are always moving, albeit very slowly. They can collide, pull apart, or slide against one another at plate boundaries.
When stress builds up at a fault line—like the famous San Andreas Fault—the energy is released as an earthquake. This release can happen suddenly, causing a main shock, or it may be preceded by smaller foreshocks. Aftershocks often follow, as the crust adjusts to the new position. The surface movement associated with these shifts can create significant changes in the landscape.
Measuring Earthquakes
Seismometers are crucial for measuring earthquakes. These sensitive instruments detect ground motion caused by seismic waves.
When an earthquake occurs, it generates waves that travel through the Earth. Seismographs record these movements on a seismogram, which provides valuable data for scientists.
The magnitude of an earthquake quantifies its size and energy release. It is measured on a logarithmic scale, often the moment magnitude scale (Mw).
The intensity, however, describes the effects of the earthquake on people, buildings, and the Earth’s surface. Understanding these measurements helps in forecasting future seismicity and assessing earthquake prediction methods.
Seismic Waves and Energy Dispersion
When an earthquake strikes, energy travels in the form of seismic waves. There are two main types: primary (P) waves and secondary (S) waves.
P waves are compressional waves that move through solids and liquids, while S waves are shear waves that can only travel through solids.
These waves spread from the earthquake’s hypocenter, the point where the earthquake originates, to the epicenter above it. The strength and speed of these waves determine how far the effects are felt.
Their interaction with different geological materials influences the intensity of shaking. Studying these waves enhances knowledge of the Earth’s interior, which includes the crust, mantle, and core layers.
Earthquake Preparedness and Impact
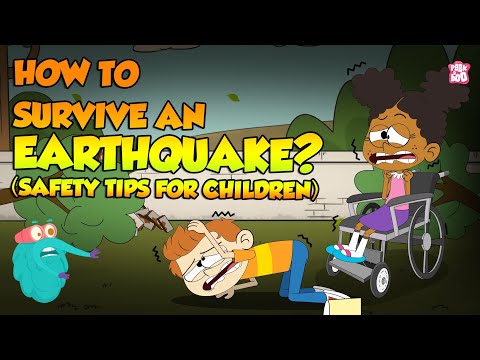
Preparedness for earthquakes involves monitoring and predicting seismic activity to minimize damage and loss of life. Understanding the economic and societal consequences of major earthquakes is crucial for developing effective response strategies.
Earthquake Monitoring and Forecasting
Earthquake monitoring utilizes a network of seismographs to measure ground movements. This data helps scientists identify seismic activity patterns, including microseismicity, which refers to small, often undetectable quakes.
Geophysicists study these patterns to enhance earthquake prediction methods.
New technologies, including artificial intelligence and machine learning, improve the accuracy of forecasts. These tools can analyze vast amounts of data quickly, identifying potential earthquake risks associated with friction and strain along fault lines.
Accurate forecasting enables communities to prepare effectively, as seen in areas like the Sendai region, where a system provides timely alerts about imminent earthquakes.
Economic and Societal Consequences
Major earthquakes have significant economic impacts.
For instance, the Northridge earthquake in 1994 caused billions in damages, demonstrating the need for robust preparedness measures.
Property destruction, business interruptions, and infrastructure damage strain local economies and can take years to recover.
The societal effects are equally serious.
Lives lost, injuries sustained, and displacement from homes create long-lasting emotional and physical scars.
Communities often rely on immediate disaster response services, which are critical in minimizing casualties.
Preparedness programs help educate citizens on safety procedures, ensuring they know how to respond during seismic events.
This proactive approach enhances resilience, helping societies rebound more swiftly after a disaster.